Biomedical optics is a broad and highly interdisciplinary field with participants from physics, biophysics, biochemistry, engineering, biology, medicine, mathematics, and computer science. This research encompasses all aspects of optical imaging and spectroscopy ranging from subcellular lengthscales to large tissue volumes such as the brain and breast. It is concerned with cancer detection & therapeutics, non-invasive tissue biopsy, functional activation & mechanisms, and the coupling between physiological responses and optical signals. My group is involved in several projects, some of which are described here.
Spectroscopy and Imaging with Diffusing Light
Many materials are visually opaque because photons traveling within them are predominantly scattered rather than absorbed. Some common examples of these highly scattering media include white paint, foam, mayonnaise, and human tissue. Indeed, anyone who has held a flashlight up to his or her hand will notice some of this light is transmitted, albeit after experiencing many scattering events. Light travels through these materials in a process similar to heat diffusion.
Diffuse light imaging and spectroscopy aims to investigate tissue physiology millimeters to centimeters below the tissue surface. The cost of this aim is we must abandon traditional optical spectroscopies and traditional microscopy. Traditional methodologies require optically thin samples. In addition, light penetration must be large in order to reach tissue located centimeters below the surface. Fortunately, a spectral window exists within tissues in the near-infrared from approximately 700 nm to 900 nm, wherein photon transport is dominated by scattering rather than absorption. The absorption of hemoglobin and water is small in the near-infrared, but elastic scattering from organelles and other microscopic interfaces is large. These are precisely the conditions required for application of the diffusion model for light transport. Using this physical model it is possible to quantitatively separate the effects of tissue scattering from tissue absorption, and to accurately incorporate the influence of boundaries, such as the air-tissue interface, into the light transport theory. The diffusion approximation also provides a tractable basis for tomographic approaches to image reconstruction using highly scattered light.
Even though absorption in the near-infrared is relatively small, the spectra of major tissue chromophores, particularly oxy- and deoxy-hemoglobin and water, differ significantly in the near-infrared. As a result, the diffuse optical methods are sensitive to blood dynamics, blood volume, blood oxygen saturation, and water and lipid content of interrogated tissues. In addition, one can induce optical contrast in tissues with exogenous contrast agents, for example chemical species that occupy vascular and extravascular space and preferentially accumulate in diseased tissue. Together these sensitivities provide experimenters with access to a wide spectrum of biophysical problems. The greater blood supply and metabolism of tumors compared to surrounding tissues provides target heterogeneity for tissue maps based on absorption. Similar maps can be applied for studies of brain bleeding, and cerebral oxygen dynamics associated with activation by mental and physical stimulation. Other applications of the deep tissue methods include the study of mitochondrial diseases, the study of muscle function and disease, and the study of photodynamic therapy.
Our laboratory has made substantive contributions to problems of light transport in highly scattering media such as tissue and to diffuse optical tomography. In early papers we demonstrated how diffusive waves in highly scattering media obey rules of optics such as refraction, interference and diffraction. We also published first experiments demonstrating tomographic reconstruction of absorption and scattering heterogeneities with diffusive waves, we were among the first groups to show how to reconstruct the lifetime and concentration of fluorophores in tissues, and we introduced the correlation diffusion equation, a methodology whereby diffused temporal light field correlation functions can be used to reconstruct heterogeneous dynamical flow properties of turbid media. We have examined the fundamental resolution and characterization limitations of diffuse optical techniques, and we have combined diffuse optical methods with other imaging modalities such as ultrasound and MRI. We continue to develop theoretical tools for DOT, for example, we have elucidated the resolution and sensitivity trade-offs associated with specific instrumental geometries and data types, we have enabled experimenters to choose optimal source wavelengths for clinical experiments, and we have clarified the conditions for which differential measurements are well suited for quantitative tomography. These and other research papers are in our list of publications. Useful review articles include papers from Physics Today (A. Yodh & B. Chance, Spectroscopy and imaging with diffusing light, Physics Today 48 (1995)) and the CRC Biomedical Photonics Handbook (A.G. Yodh & D.A. Boas, Functional imaging with diffusing light, in Biomedical Photonics Handbook , Vo-Dinh, T., Ed., CRC Press: Boca Raton. p. 21-1-21-45, (2003) )
Functional Imaging and Spectroscopy of Brain, Breast and Muscle
Our current physiological applications of optical light imaging and spectroscopy include functional imaging of brain activation and metabolism, study of muscle function and physiology, optical mammography, and characterization of tumors and tumor responses to therapy. A few of these applications are discussed below.
Some other research can be found at http://www.lrsm.upenn.edu/pmi .
Breast Cancer Imaging and Spectroscopy
The long-range goal of this research is to assess and improve the breast tumor diagnostic capabilities of diffuse optical tomography (DOT) with near infrared light. The most effective clinical role for DOT in the screening and diagnosis of breast cancer has yet to be determined. However, it is clear that DOT provides exquisite functional information directly related to tumor pathophysiology (e.g. metabolic activity, angiogenesis, and blood flow/concentration), and complementary to structural and functional information provided by conventional medical imaging. Furthermore, advances in diffuse optical tomography of breast are critical for exploitation of the advances of molecular imaging, an emerging field of medicine promising development of a new generation of optical contrast agents.
In one set of investigations, we have assembled a parallel-plate soft-compression apparatus for breast cancer imaging, and we have begun pilot clinical studies with the instrument at the Hospital of the University of Pennsylvania (HUP). On the clinical side, we are accumulating normal subjects and tumor-bearing patients in pilot studies; we have also initiated a new study to track tumor properties of patients undergoing neoadjuvant chemotherapy prior to surgery. On the basic science and engineering side, we are identifying and implementing critical hardware improvements for the instrument and software improvements for image reconstruction. These improvements will increase the speed and spectral coverage of the instrument, and will reduce image artifacts. Hand-held scanners are also being developed to track tissue blood oxygenation and blood flow in palpable tumors. The images below show an example of tumor imaging in human breast with light. Our breast cancer research programs are an integral part of a nationwide Network for Translational Research in Optical Imaging (NTROI) whose central aim is to identify and develop optical technologies for early translation to the clinic (see: NTROI at the University of California).
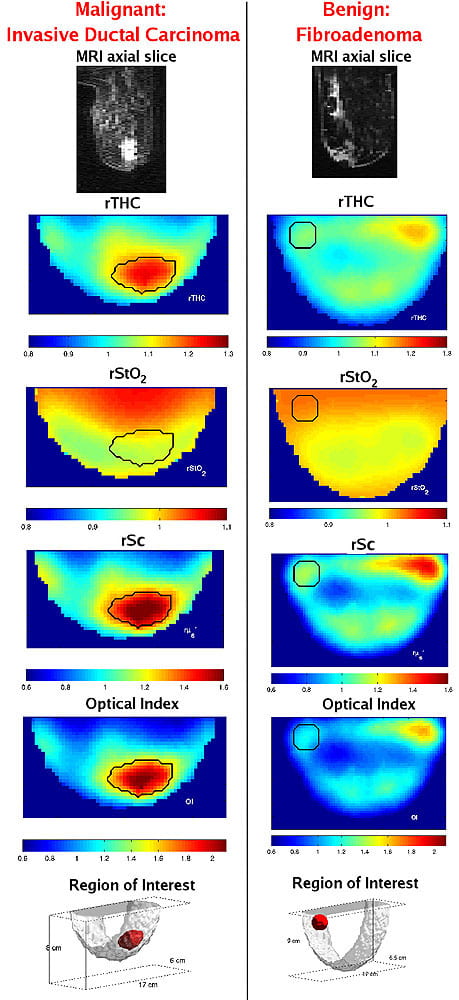
MRI axial slice, DOT axial slices of relative total hemoglobin concentration (rTHC), relative blood oxygen saturation (rStO2), relative tissue scattering (rSc), Optical Index, and a 3D image of region of interest are shown for malignant (left-side) and benign lesions (right-side). The black line indicates the tumor region.
Functional Imaging in Brain (Diffuse Optical Methods)
In this research we are concerned with measurement of the concentration, oxygenation, and flow characteristics of blood cells in the brain, ultimately human brain. Energy is required for cerebral functioning, and its conversion into a useful form is facilitated by oxygen delivered to the brain through the cerebral vasculature. Vascular and metabolic changes in the brain influence a wide range of phenomena of clinical importance and of basic scientific interest. In-situ measurements of any of these basic blood parameters provide insight about physiological phenomena, for example cerebral metabolism and functional heterogeneity, internal bleeding and ischemia. Simultaneous measurement of more than one of these quantities provides deeper insight into tissue physiology, and offers the possibility to directly probe tissue oxygen metabolism. Maps of flow, oxygenation and oxygen extraction can improve our basic understanding about how the brain works, and, on the clinical side, can potentially reduce mortality via detection and characterization of ischemic brain damage.
We have constructed versatile multi-modal all-optical imaging probes for measurement of total hemoglobin concentration, blood oxygenation, blood flow, the cerebral metabolic rate for oxygen (CMRO 2 ) and changes thereof in brain. The instrument combines two qualitatively different and complementary diffuse optical imaging modalities: (1) Diffuse Reflection Spectroscopy (DRS) for measurement of blood oxygen saturation and total hemoglobin concentration, and (2) Diffuse Correlation Spectroscopy (DCS) for measurement of blood flow. This instrumentation and its variants provide for three-dimensional imaging of physiological properties in small animal brain, and regional imaging information in human brain. The images below demonstrate detection of functional activation (i.e. finger tapping) in a specific region of the human brain, and also illustrate (see movie) three-dimensional imaging of blood flow in rat brain during cortical spreading depression.

In the experiment, a large local concentration of KCl initiates a ‘wave’ of neuronal depolarization that propagates outward from a central point and then repeats itself. Right – Images of blood flow in four parallel planes located within 3 mm of the skull.
Direct Optical Microscopy of Neuronal Activity in Animal Brain
The computational power of the brain arises from the diversity of information provided to each individual neuron by its particular and idiosyncratic connections. In visual cortex, 80% of connections are between nearby cells, and recent evidence suggests that the majority of computations are carried out through these local interactions. However, no current technology allows observation of activity in local cortical networks. Imaging techniques such as fMRI, EEG, or MEG average the activities of millions of cells across millimeters of tissue. Multi-electrode recording arrays sample clusters of cells located at significant distances from each other, and cannot reveal intracellular voltages, crucial for understanding the underlying biophysical mechanisms. We are developing novel optical microsopy devices that will allow observation and analysis of the intracellular voltages of hundreds to thousands of nearby cortical cells. Ultimately these technologies will be employed in both anesthetized and awake behaving animals to determine how local neuronal interactions govern learning in visual perception. This work is part of a collaborative interdisciplinary program (Meso-Scale Optical Brain Imaging of Perceptual Learning) funded by the Packard Foundation; collaborators include Diego Contreras, Brian Salzerg, Leif Finkel and Kwabena Boahen.
We have developed two new optical systems for imaging ongoing neural activity in the depths of the cortex. The first system (figure right) is based on a GRIN lens that is placed against the cortical surface, but focuses at depths up to a several hundred microns below the surface. Voltage-sensitive dyes are infused into the cortex, and the optical signal evoked in response to focused laser stimulation correlates precisely with the voltage in neurons imaged in a narrow focal plane. We have demonstrated that this GRIN lens system enables 3D imaging of neural activity of the in vivo cortex of anesthetized animals. The second system is a multifocal, multi-photon (two-photon) microscope. This microscope will enable us to resolve millisecond-scale activity of large populations of nearby cells to be imaged for the first time. These optical studies will take advantage of voltage sensitive dyes and the extensive intracellular electrophysiological data already obtained by our colleague, Diego Contreras.
Diffuse Optical Monitoring of Muscle
Noninvasive characterization of oxygen consumption and metabolism in skeletal muscles has important applications in exercise medicine and for understanding of vascular conditions such as peripheral arterial disease. Improved measurements of these quantities may lead to improved screening and treatment assessment, as well as to improved fundamental understanding of muscle function. The lack of portable, noninvasive technologies for continuous monitoring of both blood flow and oxygenation in the deep microcirculation capillary bed has led us to develop a hybrid instrument that monitors blood flow by effectively measuring the optical phase shifts caused by moving blood cells, and measures tissue optical properties such as absorption and scattering (and associated physiological properties such as blood oxygen saturation and total hemoglobin concentration). We have put together a program using this instrument to study blood flow and oxygenation in cuffed and exercising human muscle. Recently we have demonstrated the clinical use of all-optical methods for simultaneously measuring multiple hemodynamic parameters in muscle. These hemodynamic parameters permit estimation of changes in oxygen metabolism. Pilot studies on a single patient with peripheral arterial disease (PAD) suggest we are able to distinguish features differentiating normal and diseased responses. Multi-parameter dynamic measurements such as these are essential for the assessment of disease and for evaluating of healthy muscle oxidative metabolism. Such information has potential to improve diagnostic and treatment options for PAD patients.
Photodynamic Therapy
Photodynamic therapy (PDT) is a relatively new experimental cancer therapy that has been used with some success for the treatment of solid tumors and surface malignancies. The method requires administration of a molecular photosensitizer that localizes in tumor tissue and is subsequently activated by exposure to optical radiation. The excited photosensitizer initiates a cascade of chemical reactions, often involving highly reactive oxygen intermediates, that can produce selective tumor regression and destruction. The efficacy of PDT depends on sufficient concentrations of photons, photosensitizer and oxygen in the tumor tissue. Necrosis is thought to be brought about by a variety of mechanisms; for example, the action of irradiation-induced singlet oxygen on critical cellular components is believed to cause vascular collapse leading further to the regional breakdown of O2 and nutrient delivery.
Our program uses diffuse optical absorption spectroscopy to determine the properties of macroscopic tissue volumes, diffuse fluorescence to characterize photosensitizer distributions in macroscopic tissue volumes, and diffuse photon correlation spectroscopy to estimate tissue blood flow. On the one hand we are developing new paradigms for PDT dosimetry in the clinic, and on the other hand we are exploring the mechanisms of PDT action in animal models. One of our aims is to develop macroscopic or tissue-averaged in-situ measurements that can provide critical feedback to the clinician needed to improve PDT treatment.
Some of our most exciting recent work has been done in animal models with collaborator Theresa Busch (Radiation Oncology, PENN). This research clearly demonstrates that measurements of blood oxygen saturation and changes in tumor blood flow during PDT can be used to predict treatment outcome. In one study we utilized broadband diffuse reflectance spectroscopy to show that knowledge about changes in the blood oxygenation of a tumor within minutes after PDT can be used to predict its long-term treatment response. In another study a unique non-contact probe was developed to monitor the relative blood flow in mouse tumors during PDT. Within minutes of beginning PDT, relative blood flow rapidly increased, followed by a decline and subsequent peaks and declines associated with various flow kinetics. We found that the slope (Flow Reduction Rate) and duration (Interval Time) over which relative blood flow decreased (following the initial PDT-induced increase) was highly associated with treatment durability. This research represents one project in a large NIH funded program project originating in the Department of Radiation Oncology that is focused on PDT of Intraperitoneal Disease.